Uniform Circular Motion
Uniform circular motion can be described as the motion of an object in a circle at a constant speed. As an object moves in a circle, it is constantly changing its direction. At all instances, the object is moving tangent to the circle. Since the direction of the velocity vector is the same as the direction of the object's motion, the velocity vector is directed tangent to the circle as well. The animation at the right depicts this by means of a vector arrow.
An object moving in a circle is accelerating. Accelerating objects are objects which are changing their velocity - either the speed (i.e., magnitude of the velocity vector) or the direction. An object undergoing uniform circular motion is moving with a constant speed. Nonetheless, it is accelerating due to its change in direction. The direction of the acceleration is inwards. The animation at the right depicts this by means of a vector arrow.
The final motion characteristic for an object undergoing uniform circular motion is the net force. The net force acting upon such an object is directed towards the center of the circle. The net force is said to be an inward or centripetalforce. Without such an inward force, an object would continue in a straight line, never deviating from its direction. Yet, with the inward net force directed perpendicular to the velocity vector, the object is always changing its direction and undergoing an inward acceleration.
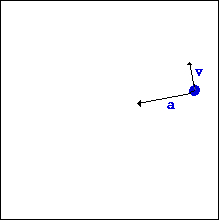
Inertia and the Right Hand Turn
Many students of physics do not believe in centripetal ("inwards") forces. Even after completion of a thorough physics course, such students will still incorrectly believe that an object moving in a circle experiences an outward force. Perhaps the reason for adhering to this misconception stems from their experiences with riding as a passenger in automobiles and amusement park rides.
Imagine that you are a passenger in a car which is making a right-hand turn. As the car begins to take the turn to the right, you often feel as though you are sliding to the left. The car is turning to the right due to the inward force, yet youfeel as though you are being forced leftward or outward. In actuality, the car is beginning its turning motion (to the right) while you continue in a straight line path. This motion can be better understood by examining the animation below.
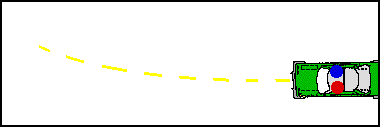
Observe in the animation that the passenger (in blue) continues in a straight-line motion for a short period of time after the car begins to make its turn. In fact, the passenger follows a straight-line path until striking the shoulder of the driver (in red). Once striking the driver, a force is applied to the passenger to force the passenger to the right and thus complete the turn.
An inward net force is required to make a turn in a circle. This inward net force requirement is known as a centripetal force requirement. In the absence of any net force, an object in motion (such as the passenger) continues in motion in a straight line at constant speed. This is Newton's first law of motion. While the car begins to make the turn, the passenger and the seat begin to edge rightward. In a sense, the car is beginning to slide out from under the passenger. Once striking the driver, the passenger can now turn with the car and experience some circle-like motion. There is never any outward force exerted upon the passenger. The passenger is either moving straight ahead in the absence of a force or moving along a circular path in the presence of an inward-directed force.
Uniform circular motion can be described as the motion of an object in a circle at a constant speed. As an object moves in a circle, it is constantly changing its direction. Because of this direction change, you can be certain that an object undergoing circular motion is accelerating (even if it is moving at constant speed). And in accord with Newton's laws of motion, an accelerating object must be acted upon by an unbalanced force. This unbalanced force is in the same direction as the direction of the acceleration. For objects in uniform circular motion, the net force and subsequent acceleration is directed inwards. Circular motion requires a net inward or "centripetal" force.
Without a net centripetal force, an object cannot travel in circular motion. In fact, if the forces are balanced, then an object in motion continues in motion in a straight line at constant speed. This can be demonstrated by carrying a tennis ball upon a flat, level board. Once the tennis ball and the board are in motion, they will continue in motion in the same direction at the same speed unless acted upon by an unbalanced force. This demonstrates Newton's first law of motion. But if an unbalanced force is applied to the flat board, then the flat board will accelerate. If the force is continually directed towards a point at the center of the circle, then the flat board will round the corner in a circular-like path. The ball on the other hand will continue to move in the same direction since there is no unbalanced force acting upon it. The board will move out from under the tennis ball. This is illustrated in the animation on the left below.
Now if a block is secured to the board in such a manner that the block applies an unbalanced force to the ball that is directed towards the center of the circle, then quite another phenomenon will be observed. With the block providing a normal force directed inward, the ball can round the corner in a circular-like path. The block supplies the centripetal force required for circular motion. With the centripetal motion requirement met, uniform circular motion can occur. This is illustrated in the animation on the right.
Without a centripetal force, an object in motion continues along a straight-line path. With a centripetal force, an object in motion will be accelerated and change its direction.
The tendency of a moving object to continue in a straight line in the absence of an unbalanced force and to turn in a circle in the presence of a inward-directed force (i.e., centripetal force) is a common experience as a passenger in an automobile. When the car makes a sudden turn, the passengers tend to continue in their straight line path. This straight line motion continues until the presence of a side door or another passenger pushes upon the passenger in order to accelerate him/her towards the center of the turn. The force experienced by the passenger is an inward force; without it, the passenger would slide out of the car.
Roller Coaster G-Forces
Roller coaster rides are notorious for creating accelerations and g-forces which are capable of transforming stomach contents into airborne projectiles. As a rider starts the descent down the first drop, she begins a one-minute adventure filled with various sensations of weightlessness, heaviness, and jerkiness. The parts of the ride which are most responsible for these sensations of weightlessness and heaviness are the clothoid loops. The explanation for the various sensations experienced on a roller coaster loop are associated with Newton's laws of motion and the physics of circular motion.
A clothoid loop has a constantly curving shape with sections which resemble the curve of a circle (in actuality, it is considered to be a section of a cornu spiral having a constantly changing radius). A coaster rider is continuously altering her direction of motion while moving through the loop. At all times, the direction of motion could be described as being tangent to the loop. This change in direction is caused by the presence of unbalanced forces and results in an acceleration. Not only is there an acceleration, the magnitude and direction of the acceleration is continuously changing. Within nearly a one second time interval, the riders may experience accelerations of 20 m/s/s downwards to 30 m/s/s upwards; such drastic changes in acceleration normally occur as the rider moves from the top of the loop to the bottom of the loop. These drastic changes in accelerations are the cause of much of the thrill (and the occasionally dizziness) experienced by coaster riders.
To understand the feelings of weightlessness and heaviness experienced while riding through a loop, it is important to think about the forces acting upon the riders. To simplify the discussion, we will assume that there are negligible amounts of air resistance acting upon the riders. Thus, the only forces exerted upon the riders are the force of gravity and the normal force (the force of the seat pushing up on the rider). The force of gravity is at all times directed downwards and the normal force is at all times directed perpendicular to the seat of the car. Since the orientation of the car on the track is continuously changing, the normal force is continuously changing its direction. The magnitude and direction of these two forces during the motion through the loop are depicted in the animation below.
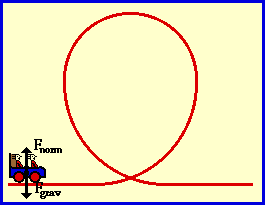
For an object to move along a circular path at a constant speed, there must be a net inward force acting upon the rider. This is commonly referred to as the centripetal force requirement. The motion through a coaster loop isn't precisely an example of moving in a circle at constant speed since the loop is neither circular not the speed constant. Nonetheless, because of the similarity of the motion along the loop's path to uniform circular motion, principles of uniform circular motion can be applied to the rider. The net force acting upon the rider has an inwards direction (towards the center of the circle). Since the net force is the vector sum of all the forces, the head-to-tail addition of the normal force and the gravity force should sum to a resultant force which points inward. The diagram below depicts the free-body diagrams for a rider at four locations along the loop. The diagram also shows that the vector sum of the two forces (i.e., the net force) points mostly towards the center of the loop for each of the locations.
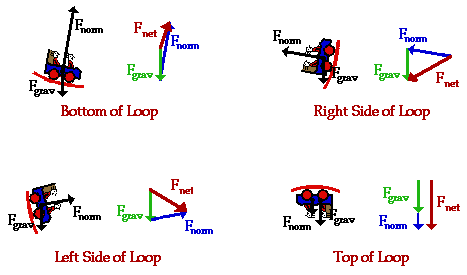
Feelings of weightlessness and heaviness are associated with the normal force; they have little to do with the force of gravity. A person who feels weightless has not lost weight. The force of gravity acting upon the person is the same magnitude as it always is. Observe that in the animation above the force of gravity is everywhere the same. The normal force however has a small magnitude at the top of the loop (where the rider often feels weightless) and a large magnitude at the bottom of the loop (where the rider often feels heavy). The normal force is large at the bottom of the loop because in order for the net force to be directed inward, the normal force must be greater than theoutward gravity force. At the top of the loop, the gravity force is directed inward and thus, there is no need for a large normal force in order to sustain the circular motion. The fact that a rider experiences a large force exerted by the seat upon her body when at the bottom of the loop is the explanation of why she feels heavy. In actuality, she is notheavier; she is only experiencing the large magnitude of force which is normally exerted by seats upon heavy people while at rest.

Satellite Motion
A satellite is often thought of as being a projectile which is orbiting the Earth. But how can a projectile orbit the Earth? Doesn't a projectile accelerate towards the Earth under the influence of gravity? And as such, wouldn't any projectile ultimately fall towards the Earth and collide with the Earth, thus ceasing its orbit?
These are all good questions and represent stumbling blocks for many students of physics. We will discuss each question here. First, an orbiting satellite is a projectile in the sense that the only force acting upon an orbiting satellite is the force of gravity. Most Earth-orbiting satellites are orbiting at a distance high above the Earth such that their motion is unaffected by forces of air resistance. Indeed, a
satellite is a projectile.
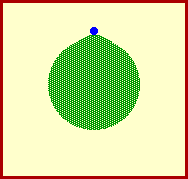
Second, a satellite is acted upon by the force of gravity and this force does accelerate it towards the Earth. In the absence of gravity a satellite would move in a straight line path tangent to the Earth. In the absence of any forces whatsoever, an object in motion (such as a satellite) would continue in motion with the same speed and in the same direction. This is the law of inertia. The force of gravity acts upon a high speed satellite to deviate its trajectory from a straight-line inertial path. Indeed, a satellite is accelerating towards the Earth due to the force of gravity.
Finally, a satellite does fall towards the Earth; only it never falls into the Earth. To understand this concept, we have to remind ourselves of the fact that the Earth is round; that is the Earth curves. In fact, scientists know that on average, the Earth curves approximately 5 meters downward for every 8000 meters along its horizon. If you were to look out horizontally along the horizon of the Earth for 8000 meters, you would observe that the Earth curves downwards below this straight-line path a distance of 5 meters. In order for a satellite to successfully orbit the Earth, it must travel a horizontal distance of 8000 meters before falling a vertical distance of 5 meters. A horizontally launched projectile falls a vertical distance of 5 meters in its first second of motion. To avoid hitting the Earth, an orbiting projectile must be launched with a horizontal speed of 8000 m/s. When launched at this speed, the projectile will fall towards the Earth with a trajectory which matches the curvature of the Earth. As
such, the projectile will fall around the Earth, always accelerating towards it under the influence of gravity, yet never colliding into it since the Earth is constantly curving at the same rate. Such a projectile is an orbiting satellite.
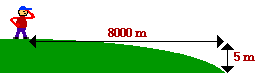
To further understanding the concept of a projectile orbiting around the Earth, consider the following thought experiment. Suppose that a very powerful cannon was mounted on top of a very tall mountain. Suppose that the mountain was so tall that any object set in motion from the mountaintop would be unaffected by air drag. Suppose that several cannonballs were fired from the cannon at various speeds - say speeds of 8000 m/s, less than 8000 m/s, and more than 8000 m/s. A cannonball launched with speeds less than 8000 m/s would eventually fall to the Earth. A cannonball launched with a speed of 8000 m/s would orbit the Earth in a circular path. Finally, a cannonball launched with a speed greater than 8000 m/s would orbit the Earth in an elliptical path. The animations below depict these ideas.
Projectile falls to Earth ![]() | Projectile falls to Earth ![]() |
Projectile orbits Earth - Circular Path ![]() | Projectile orbits Earth - Elliptical Path ![]() |
Two final notes should be made about satellite motion. First, the 8000 m/s figure used in the above discussion applies to satellites launched from heights just above Earth's surface. Since gravitational influences decrease with the height above the Earth, the orbital speed required for a circular orbit is less than 8000 m/s at significantly greater heights above Earth's surface.
Second, there is an upper limit on the orbital speed of a satellite. If launched with too great of a speed, a projectile will escape Earth's gravitational influences and continue in motion without actually orbiting the Earth. Such a projectile will continue in motion until influenced by the gravitational influences of other celestial bodies.
Kepler's Second Law
After studying a wealth of planetary data for the motion of the planets about the sun, Johannes Kepler proposed three laws of planetary motion. Kepler's second law states
An imaginary line joining a planet and the sun sweeps out an equal area of space in equal amounts of time.
The animation below depicts the elliptical orbit of a planet about the sun.
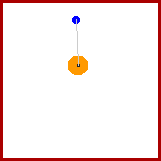
The dot pattern shows that as the planet is closest the sun, the planet is moving fastest and as the planet is farthest from the sun, it is moving slowest. Nonetheless, the imaginary line joining the center of the planet to the center of the sun sweeps out the same amount of area in each equal interval of time.
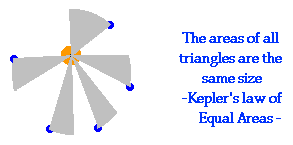

No comments:
Post a Comment